What is steam
Steam is an invisible gas consisting of vaporized water, which is formed when water boils. When steam is visible, it contains the visible mist of water droplets. Such steam is referred to as “wet steam“, but “dry steam” is always invisible. At lower pressures, such as in the upper atmosphere or in the condenser of thermal power plants, steam can exist at a lower temperature than the nominal 100 °C at standard temperature and pressure.
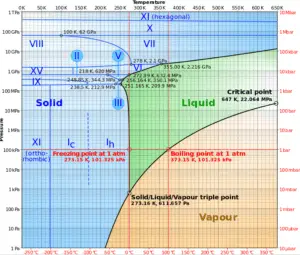
Source: wikipedia.org CC BY-SA
Since water and steam are a common media used for heat exchange and for conversion of energy, steam is generated on a large scale by energy systems, as, for example, in thermal power plants. As is typical in all conventional thermal power plants the heat is used to generate steam which drives a steam turbine connected to a generator which produces electricity. Note that, modern steam turbines are used to generate more than 80% of the world’s electricity.
Steam is generally categorized according to the vapor/(liquid + vapor) fraction. This fraction is very important parameter of steam and it is known as the vapor quality.
See also: Properties of Water
Vapor Quality – Dryness Fraction
As can be seen from the phase diagram of water, in the two-phase regions (e.g. on the border of vapor/liquid phases), specifying temperature alone will set the pressure and specifying pressure will set the temperature. But these parameters will not define the volume and enthalpy because we will need to know the relative proportion of the two phases present.
The mass fraction of the vapor in a two-phase liquid-vapor region is called the vapor quality (or dryness fraction), x, and it is given by following formula:
The value of the quality ranges from zero to unity. Although defined as a ratio, the quality is frequently given as a percentage. From this point of view, we distinguish between three basic types of steam. It must be added, at x=0, we are talking about saturated liquid state (single-phase).
This classification of steam has its limitation. Consider the behavior of the system which is heated at the pressure, that is higher than the critical pressure. In this case, there would be no change in phase from liquid to steam. At all states there would be only one phase. Vaporization and condensation can occur only when the pressure is less than the critical pressure. The terms liquid and vapor tend to lose their significance. At pressure, that is higher than the critical pressure, water is in special state, that is known as supercritical fluid state.
See also: Saturation
See also: Supercritical Fluid
See also: Throttling of Steam
Properties of Steam – Steam Tables
Water and steam are a common fluid used for heat exchange in the primary circuit (from surface of fuel rods to the coolant flow) and in the secondary circuit. It used due to its availability and high heat capacity, both for cooling and heating. It is especially effective to transport heat through vaporization and condensation of water because of its very large latent heat of vaporization.
A disadvantage is that water moderated reactors have to use high pressure primary circuit in order to keep water in liquid state and in order to achieve sufficient thermodynamic efficiency. Water and steam also reacts with metals commonly found in industries such as steel and copper that are oxidized faster by untreated water and steam. In almost all thermal power stations (coal, gas, nuclear), water is used as the working fluid (used in a closed loop between boiler, steam turbine and condenser), and the coolant (used to exchange the waste heat to a water body or carry it away by evaporation in a cooling tower).
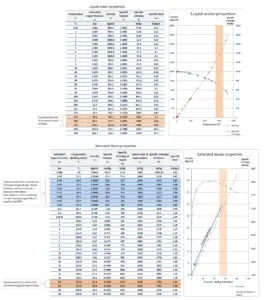
Water and steam are a common medium because their properties are very well known. Their properties are tabulated in so called “Steam Tables”. In these tables the basic and key properties, such as pressure, temperature, enthalpy, density and specific heat, are tabulated along the vapor-liquid saturation curve as a function of both temperature and pressure. The properties are also tabulated for single-phase states (compressed water or superheated steam) on a grid of temperatures and pressures extending to 2000 ºC and 1000 MPa.
Further comprehensive authoritative data can be found at the NIST Webbook page on thermophysical properties of fluids.
See also: Steam Tables
Special Reference: Allan H. Harvey. Thermodynamic Properties of Water, NISTIR 5078. Retrieved from https://www.nist.gov/sites/default/files/documents/srd/NISTIR5078.htm
Examples
We hope, this article, Steam – Properties of Steam, helps you. If so, give us a like in the sidebar. Main purpose of this website is to help the public to learn some interesting and important information about thermal engineering.